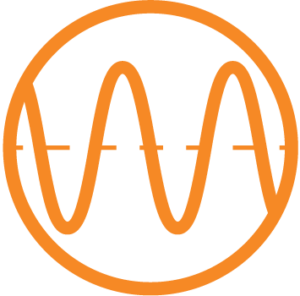
Atomic GHz/THz Sensor & Imager
Goals and background
Calibration-free sensing and vector imaging of microwave and THz fields sustain a large range of applications, for which atomic vapor cells have shown to be a powerful tool. In macQsimal we were using atoms to image high-frequency electromagnetic fields in the gigahertz (GHz) and terahertz (THz) frequency range.
Partners from UNIBAS demonstrated a microwaves magnetic near-field imaging technique based on frequency domain Rabi spectroscopy, using a compact MEMS atomic vapor cell as an imaging sensor.
The principle of THz imaging used by researchers from UDUR was based on the use of Rydberg atoms to perform THz-to-optical frequency conversion, meaning converting difficult-to-detect THz radiation into easy-to-detect optical photons. The vapour-cell-based technique for THz imaging holds the advantage of high speed and sensitivity, low cost and easy operation with the avenues of broad applications.
The macQsimal partners collaborated closely to design and develop an atomic GHz imaging device demonstration and portable Rydberg atomic THz imaging system.
Key results and impact
- An atomic GHz imaging device demonstrator operating with MEMS vapr cells was built, tested and validated
- Atomic spectrum analyzer operation mode was demonstrated and identified as the most relevant and promising for applications in the microwave communications industry
- A Rydberg-based THz imager was demonstrated, tested and validated, showing high sensitivity, bandwidth, and spatial resolution
- Calibrated THz electric field sensing was demonstrated providing a new tool for metrology in the terahertz frequency range
Outcomes
- Terahertz electrometry via infrared spectroscopy of atomic vapor
Chen, S., Reed, D. J., MacKellar, A. R., Downes, L. A., Almuhawish, N. F. A., Jamieson, M. J., Adams, C. S., Weatherill, K. J. (2022) Optica, 9(5), 485-491. - Full-Field Terahertz Imaging at Kilohertz Frame Rates Using Atomic Vapor
Downes, L.A., MacKellar, A.R., Whiting, D.J., Bourgenot, C., Adams, C.S., Weatherill, K.J. (2020) Physical Review X, 10(1), 011027. A preprint of this publication is available on arXiv - Narrowband photon pairs with independent frequency tuning for quantum light-matter interactions
Prakash, V., Bianchet, L.C., Cuairan, M.T., Gomez, P., Bruno, N., Mitchell, M.W. (2019) Optics Express, 27(26), 38463-38478.
The macQsimal partners produced seven deliverables on GHz/THz sensors & imagers. Please refer to the publicly available deliverables for more details.
- Microwave magnetic field imaging based on frequency-domain Rabi spectroscopy with an ultrathin atomic vapor cell UNIBAS, 2021, 2nd EQTC, online.
- Towards a frequency-tunable microwave magnetic field imager with ultrathin atomic vapor cells UNIBAS, 2020, micro-WOPM 2020, online.
- GHz microwave field imaging with atomic vapor cells UNIBAS, 2019, Workshop on optically-pumped magnetometers, Mainz.
Performed activities
Establishing an imaging principle
The principle of THz imaging here is to use Rydberg atoms to perform THz-to-optical frequency conversion, converting difficult-to-detect THz radiation into easy-to-detect optical photons. The electric dipole transitions between neighboring Rydberg states of alkali atoms lie in the THz frequency range. These transitions have very large dipole moments, resulting in a high probability of interaction with a resonant THz field. When THz radiates the cell, there is bright visible fluorescence generated from the cell. We use the emitted visible photons to create an image of the incident THz field in a single exposure. While some imaging systems raster their detector to capture the image pixel-by-pixel in sequence, our system captures all pixels in a 2D image simultaneously, increasing our image acquisition speed. The versatility of this approach means that any optical camera can be employed and can easily be substituted into the system.
Cell closeup and THz fluorescence
We used a cuboid quartz cell filled with alkali atoms to image THz. The atoms are firstly excited to a certain Rydberg state. When the THz is on, there is a bright green fluorescence radiated from the cell. This bright fluorescence image can be captured by an optical camera.
THz waves are able to penetrate most wrapper materials, such as plastic and cardboard. Therefore, THz imaging bares great potential in the food industry, as it enables quality and security checks without the necessity to open food wrappers. In macQsimal, we demonstrated this feature of THz waves on a hazelnut chocolate bar. The resulting image is visualised on the top right and revealed the hazelnut distribution within the chocolate bar. This technique may be used to discriminate food contaminants and monitor food quality and thus be widely implemented in the food production line of the future.
THz imaging and laboratory setup
As different atoms enable different THz transitions and THz fluorescence colors, the project partners of DURHAM implemented both, the Rb imaging system (left) and Cs imaging system (right), to perform their research. On each table, the 3 lasers, together with their locking systems to excite the alkali atoms into a Rydberg state sensitive to the THz frequency of interest, as well as the THz source and the atomic cell, were installed.
Towards market applicability
The beneficiaries UNIBAS, UDUR and STUTT have collaborated on the development of an atomic GHz field imaging device demonstrator. This device was designed to operate in two modes: as a GHz field imager and an atomic spectrum analyzer. The GHz field imager has demonstrated the capability to detect microwave signals at a power of approx. -50 dBm.The analyzer mode has been identified as the most relevant and promising for applications in the microwave communications industry.
The aim of this setup was to analyze the spatial distribution of the radiofrequency (RF) field emitted by an electrical device, such as a waveguide or an antenna. The electrical device is brought to close proximity to the cell so that its RF field interacts with the Rubidium atoms. If the frequency of the RF field matches the resonant frequency of the Rubidium atoms, the atoms absorb the laser light in regions where a strong field is present. As a result, the RF field distribution is directly imprinted on the camera image.
The novel approach for the spectrum analysis aimed to broadband it in a magnetic field gradient. A magnetic field gradient is generated by removing one of the magnets in the imaging setup. In this configuration, the vapor cell effectively performs a Fourier transform of the radiofrequency (RF) field, turning the setup into an RF spectrum analyzer.
In contrast to conventional “sweep”-based spectrum analyzers, this technique measures the entire spectrum simultaneously, because each row of pixels in the camera image corresponds to one particular frequency bin of the RF spectrum. As such, it is feasible to reach tens of GHz of instantaneous bandwidth, only limited by the magnetic field gradient of the magnet configuration. After the completion of the project, the partners from UNIBAS are continuing the development of this mode in collaboration with Rohde & Schwartz, who served as industry advisors in macQsimal.